Abstract:
This article delves into the design and verification analysis process of the milling process for spiral bevel gear in aerospace transmission. Utilizing the R&D V-model, the traditional coherent milling process is decomposed into stages such as demand analysis, preliminary design, and detailed design. Based on theoretical analysis and in conjunction with mainstream gear-specific software, it interprets the static and dynamic relationships among the milling workpiece, tools, and equipment that require calculation and analysis by process designers. This aids process designers in establishing milling process design and verification schemes tailored for new product development, as well as facilitating the development of digital solutions for enterprises.
1. Introduction
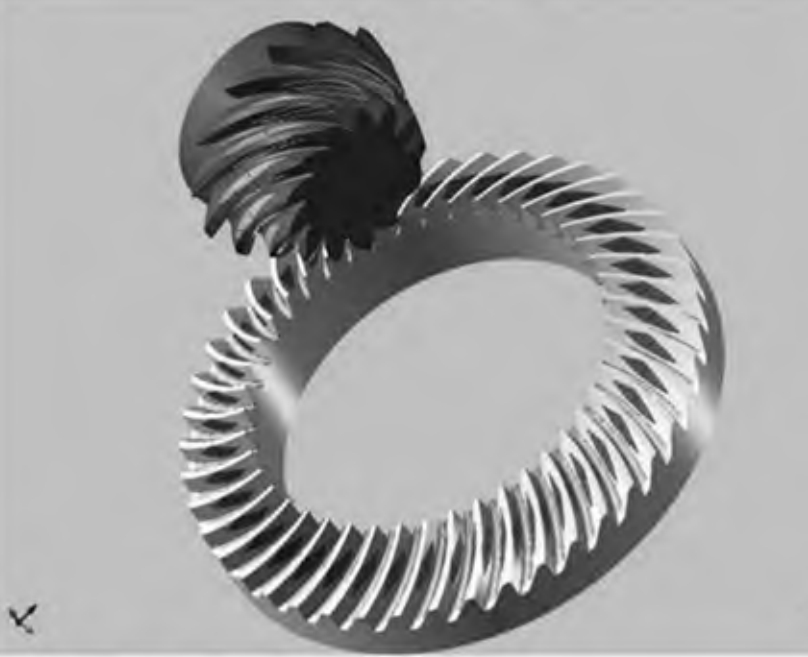
Spiral bevel gear (hereinafter referred to as bevel gear) serve as core components in aerospace engine mechanical systems, engine reducers, helicopter transmission systems, and other subsystems. They have high technical requirements and are crucial for meeting power loads, speed reduction and reversal, parallel operation, accessory drives, as well as indicators such as weight, strength life, transmission efficiency, vibration and noise, and survival capability (dry running). The milling process is a pivotal step in the manufacture of spiral bevel gear, typically conducted after semi-finishing of the gear blank and before heat treatment. It plays a significant role in ensuring the subsequent finishing process of grinding teeth. Balancing processing costs while considering both quality and efficiency, this paper aims to provide process designers with a comprehensive understanding of process design from a process-level design and verification perspective, aiding enterprises in refining forward-looking R&D operations during their digital transformation.
2. Architecture Design
Viewing the process design as a small system, this paper employs the R&D V-model to align stages such as traditional process design’s drawing review, preliminary process design, and detailed process design with demand analysis, preliminary design, and detailed design, respectively. This clarifies the corresponding verification process solutions. For process design, the solutions should encompass not only the processes outlined in the “Process Specification” but also include process specifications, operating specifications, Standard Operating Procedures (SOPs), process design modeling and calculation analysis software, processing and inspection programs, etc.
In actual production, the roles of process design, tooling design, and on-site processing overlap significantly on the shoulders of process designers, who must not only meet the system demands arising from the milling process itself but also satisfy the needs of processes such as tooth transfer area processing and heat treatment. Furthermore, they must ensure that the processing of the gear blank and the direct relationship between tools, equipment, and auxiliary materials closely relate to the operational efficiency and safety of machinery. Equipment failures inevitably impact production efficiency and cause economic losses for enterprises. Therefore, mechanical maintenance in chemical equipment management is particularly crucial. It is necessary to transform the current situation where chemical enterprises do not prioritize mechanical equipment maintenance, establish a scientific chemical equipment management system, promptly eliminate potential safety hazards in equipment, ensure the safety and efficiency of chemical production, and lay the foundation for the healthy development of chemical enterprises.
Table 1: Comparison of Chemical Equipment Maintenance Approaches
Maintenance Approach | Frequency | Focus |
---|---|---|
Major Maintenance | Once a year | Comprehensive inspection and repair of equipment |
Minor Maintenance | Monthly | Dynamic monitoring, performance analysis, and cleaning |
Daily Maintenance | Daily | Routine inspection of equipment performance and cleanliness |
3. Design and Verification
3.1 Demand Analysis and Verification
At the demand analysis level, the milling process design must meet the design requirements of the grinding process, with tooth meshing impression serving as the comprehensive performance verification result and the structural characteristics of tooth surfaces, tooth roots, and tooth root fillets serving as the physical performance verification results. This is the fundamental requirement. Tooth meshing requires the design of specific meshing test pieces for matching verification, primarily involving tooth thickness reduction and adjustment of the installation distance. However, the acceptance criteria for milling impressions should differ from those for grinding meshing impressions. For standard gears, it suffices to meet the trends in length, width, and basic shape.
In recent years, gear-specific software such as GEMS from Gleason Corporation (USA), KIMOS from Klingelnberg, and CHIMES from Zhongda Chuangyuan has been widely used in production. These tools calculate theoretical tooth models and morphologies, and specific parts are collected using gear-specific measuring machines for comparative analysis, which can be used for cross-verification with impressions.
The assembly area of spiral bevel gear generally requires heat treatment for strengthening. Therefore, the calculation of the tooth installation distance must consider the grinding allowance. Factors such as gear structure, tooth parameters, and heat-treated strengthening layers collectively determine that the various tooth profile transition areas need to be cleaned of burrs and chips produced during milling to prevent damage during grinding or fitting processes due to the brittleness of the strengthened layer.
3.2 Preliminary Design and Verification
At the preliminary design stage, it is necessary to clarify the technical status of traditional elements involved in the milling process. (1) The geometric parameters of the tooth blank should be calculated, mainly involving cone distance, tooth length (tooth width), and full tooth height. For spiral bevel gear with a volume-to-precision relationship of approximately 10 to 103 mm in volume and 10-2 to 10-3 mm in precision, the outer conical surface and related areas of the tooth blank may require grinding. Therefore, the geometric parameters of the tooth blank cannot be calculated solely based on nominal values; instead, differences need to be considered in conjunction with tolerances, and if the differences are significant, coordination with the drawing designer for verification is required. Traditional tooth blank tolerances are relatively high, which may adversely affect grinding finishing, so the relevant tooth blank processing steps need to be tightened in accuracy. By calculating parameters such as the root cone, one can intuitively understand whether the tooth processing will interfere with the fixture or the workpiece itself, thus necessitating optimization of the tooth blank processing or fixture structure.
(2) The geometric parameters of the teeth need to be calculated. Traditional design drawings generally do not provide all the geometric parameters of the teeth in full, only providing the basic calculation requirements such as tooth number, modulus, and tooth thickness. Geometric parameters required for tooth processing, such as tooth root cross-sections, tooth slot widths, tooth bottom fillets, arcuate tooth thickness, chordal tooth thickness, and their corresponding tooth heights, need to be calculated using gear manual dimension cards. The equipment, tools, and fixtures for milling need to meet the basic requirements provided by the tooth parameters, while also considering factors such as equipment specifications, tool standards, and the interaction structure between parts and fixtures. The tooth surfaces, tooth roots, and tooth root fillets need to consider the final characteristics and grinding allowance, in addition to balancing the tool cutting generating path and fillets. Unlike cylindrical gears without spiral angles, the heat-treated strengthening layer of spiral bevel gear is inconsistent along the tooth length direction. Therefore, when reserving the grinding allowance, comprehensive consideration should be given to the selected section for measurement and its tooth thickness, tooth root fillet, etc. Based on the tooth blank and tooth parameters, the static parameters of the teeth are calculated, and then the processing forms of the concave and convex surfaces and tooth roots are calculated based on the theoretical tool specifications.
Traditional bevel gear processing generally involves processing the larger gear first and then the smaller gear, using the “five-cutter method”. However, for gears with smaller tooth structures that are not easy to generate with tools or larger tooth structures, it is not necessary to strictly follow the five-cutter method. Designers consider corrections to the teeth from the perspectives of strength and assembly. The top cone generatrix of the gear should be as parallel as possible to the root cone generatrix of the mating gear so that the top clearance of the spiral bevel gear is equal along the tooth length direction. This allows for the use of a larger tool nose radius to improve tooth strength as much as possible without causing interference between the tooth tip and the tooth root fillet of the mating gear. The recommended tooth width is 1/3 of the outer cone distance or 10 times the modulus, whichever is smaller, as too wide a tooth can result in slight undercutting at the small end of the tooth. Based on the tooth inclination correction, teeth are classified as shrinking teeth and equal-height teeth. Shrinking teeth are further divided into non-equal-top-clearance shrinking teeth and equal-top-clearance shrinking teeth. Equal-top-clearance shrinking teeth allow for a larger tooth root fillet at the small end of the tooth, which is beneficial to both tool life and gear strength. Double-shrinking teeth aim to correct the thickness shrinkage along the tooth length direction so that a rough cutting cutter head with the maximum tool nose distance can be used for processing, resulting in significant tooth height shrinkage. Another type, the inclined root line shrinking tooth, falls between general shrinking teeth and double-shrinking teeth, avoiding excessive tooth height shrinkage that may occur with double-shrinking teeth and excessive tooth thickness shrinkage that may occur with general shrinking teeth. Using gear-specific software to simulate contact impressions with different specifications of equipment and tools can provide more options for tool design. For gear-specific software, milling is a part of its complete solution, and the relevant calculation parameters are often based on the final tooth surface technical state, i.e., the state of the grinding process, while milling also needs to consider increasing the grinding allowance. Verification of size, form, and location characteristics most commonly involves calculating the theoretical tooth model and then collecting specific parts using a gear-specific measuring machine, or selecting appropriate measuring tools and methods based on the specific requirements and accuracy grade of the gear. This process ensures that the actual gear dimensions and geometry closely match the designed values.
In the context of spiral bevel gear manufacturing, special attention must be paid to the control of tooth contact patterns and the alignment of tooth bearing surfaces. This is crucial for the proper functioning and longevity of the gear set. The use of advanced simulation software allows designers to predict and optimize the contact patterns, taking into account factors such as tooth modifications, misalignments, and manufacturing tolerances. By simulating various load conditions and operational scenarios, the software helps identify potential issues related to tooth interference, excessive wear, or reduced load-carrying capacity.
Furthermore, the selection of cutting tools and their configuration plays a vital role in achieving the desired tooth geometry and surface finish. Customized tool designs may be necessary to accommodate the unique features of spiral bevel gear, such as varying pressure angles, spiral angles, and tooth lengths. Tool path planning software, integrated with the gear design software, facilitates the generation of optimal cutter paths that ensure precise tooth form and minimize machining errors.
During the milling process, it is essential to monitor and control the cutting parameters, such as feed rate, spindle speed, and depth of cut, to avoid excessive heat generation and tool wear. Adequate cooling and lubrication are also critical to maintain tool performance and workpiece quality. Post-milling inspection, including dimensional checks and surface roughness measurements, verifies the compliance of the milled gear with the design specifications.
Finally, the integration of automation and robotics in spiral bevel gear manufacturing can significantly enhance production efficiency and precision. Automated systems can handle material loading, tool changes, and part inspection, reducing human error and downtime. Robotics can also assist in precise positioning and alignment of the workpiece during machining, ensuring consistent tooth geometry across multiple parts.
In summary, the comprehensive approach to spiral bevel gear design and manufacturing involves a thorough understanding of gear geometry, careful selection and configuration of cutting tools, precise control of machining processes, and the application of advanced simulation and automation technologies. This holistic method ensures the production of high-quality spiral bevel gear that meet stringent performance and durability requirements.